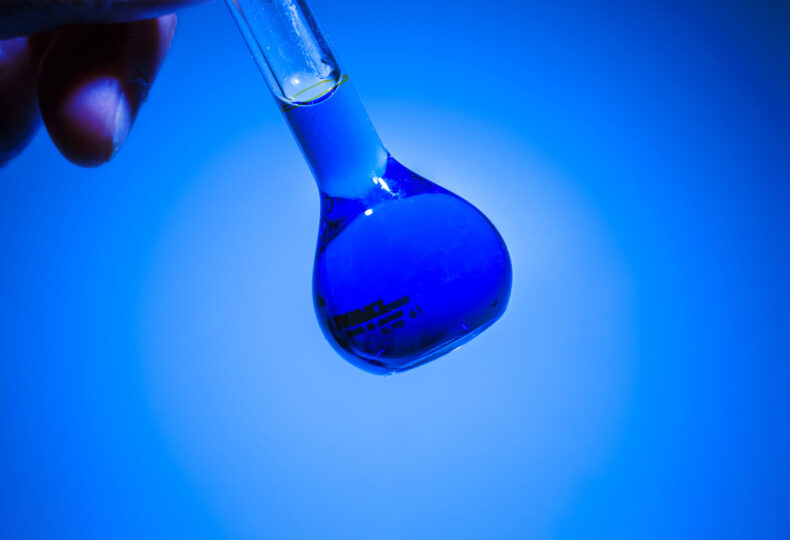
Alkene and alkyne hydroiodination, active aromatic compound iodination: Iodination reactions using alkali iodides (1): Discussion series on bromination/iodination reactions 24
In this series, we discuss bromination and iodination reactions, specialties of MANAC.
Bromides produced with brominating agents such as N-bromo compounds are used as intermediates in many fields, including pharmaceuticals and semiconductors. Equally important to these industries as bromides are iodides. Continuing with this issue, we will look closely at iodination reactions, which are utilized to create iodides.
Let’s go to the next step by looking into iodination reactions that use alkali iodides, often found as sodium iodide and potassium iodide. Owing to relatively low costs and great ease of use, alkali iodides are commonly used in many applications as reagents to synthesize iodides. Knowledge of alkali iodides is nearly indispensable when handling iodination reactions.
In this article, we will start by explaining the characteristics of sodium iodide, potassium iodide, and lithium iodide, which are common alkali iodides. Following these explanations, we will then cover iodination reactions that use alkali iodides. Be sure to use the information in this article to deepen your knowledge of alkali iodides.
contents
Characteristics of common alkali iodides
While sodium iodide and potassium iodide are most often used for general synthesis in laboratories, lithium iodide is used when the coordinating effect of metal ions is expected.
Below are the characteristics of major alkali iodides.
Sodium iodide (NaI)
Properties and applications
Sodium iodide (NaI) is a compound with a molecular weight of 149.894 g/mol (I: 84.66%) that forms white, deliquescent crystals. The compound has a melting point of 661–662°C, a boiling point of 1,304°C, and a density of 3.667 g/cm3. NaI has a salty, slightly bitter taste.
NaI is characterized by its markedly high solubility in water and its considerable solubility in alcohol, glycerin, acetone, and liquid ammonia. When precipitated from an aqueous solution of over 65°C, it forms an anhydrous salt. However, crystallization at room temperature creates a dihydrate salt (density: 2.448 g/cm3).
In addition to its use as an iodinating agent, sodium iodide is also used to treat conditions such as iodine deficiency and Graves’ disease. Recently, sodium iodide is even starting to be used in place of silver iodide for cloud seeding.
Industrial-grade chemicals can be purchased from companies such as GODO SHIGEN Co., Ltd., a MANAC partner in the iodine field.
Precautions
When left in open air, sodium iodide oxidizes and gradually takes on a yellow tinge. Add up to 1 percent ammonium hypophosphite to avoid this yellowing.
Reacting sodium iodide with a strong acid will generate hydrogen iodide, a hazardous material. Further, reacting the compound with an oxidant will release highly corrosive iodine. Therefore, caution is necessary to ensure that sodium iodide does not come into contact with strong acids or with oxidants.
Sodium iodide has an acute toxicity of LDLo 4,340 mg/kg (rat/oral) and 1,060 mg/kg (rat/intravenous). Sodium iodide irritates the eyes, mucous membranes, and skin, but is quickly excreted by the kidneys when the compound enters the body.
Potassium iodide (KI)
Properties and applications
Potassium iodide (KI) has a molecular weight of 166.02 g/mol (I: 76.45%) and presents as white, hygroscopic crystals. The melting point sits at 681–682°C with a boiling point of 1,330°C and a density of 3.130 g/cm3. In Japan, the compound is also known by its nicknames “yodo-kari,” derived from the German “jodkalium,” and “yo-potsu,” which corresponds to the Japanese Kanji characters for “iodine” and “potassium.” Potassium iodide has a salty, slightly bitter taste.
KI dissolves easily in water and fairly easily in alcohol, acetone, and liquid ammonia, while exhibiting poor solubility in ether. Thoroughly dissolving potassium iodide with iodine in an aqueous solution will generate potassium triiodide (KI3).
As a pharmacopeia drug, potassium iodide is taken as an expectorant, diuretic, and for hyperthyroidism. Potassium iodide is also used to make potassium iodide starch paper by dissolving potassium iodide and solubilized starch in water at a 3:5 ratio by weight, immersing filter paper into the solution, and then drying. Potassium iodide starch paper is used to detect trace amounts of oxidants (note that it may not effectively detect strong oxidants in some instances).
Industrial-grade potassium iodide can be purchased from companies such as GODO SHIGEN Co., Ltd.
Precautions
Similar to sodium iodide, the action of strong acids on potassium iodide will produce hydrogen iodide, and oxidants will cause the release of highly corrosive iodine. It is crucial to prevent contact between these substances.
Potassium iodide has an acute toxicity of LDLo 910/1,860 mg/kg (mouse/rabbit, oral), 1,120 mg/kg (mouse/intraperitoneal), 120 mg/kg (rat/intravenous), and a fish toxicity of LD50 3,200mg/L (rainbow trout/five days). The fine powder of potassium iodide will irritate the eyes, mucous membranes, and skin, so it is vital to prevent inhalation and contact as much as possible.
Lithium iodide
Properties and applications
The lithium iodide (LiI) compound has a molecular weight of 133.84 g/mol and forms white crystals. Its melting point is 446°C, its density is 3.890 g/cm3, and it easily dissolves in alcohol and ether catalysts, even at low temperatures.
Lithium iodide is also used as an iodinating reagent when the coordinating effect of lithium ions is expected to provide stereocontrol. For example, Ma et al. reported that when reacting allyl propiolate or propargyl propiolate with lithium iodide in acetic acid, Li+ ions are coordinated to carbonyl oxygen during the reaction, resulting in products that are regio- and stereoselectively hydroiodinated at the triple bond of the propiolate moiety2). However, because lithium iodide is considerably costly as an iodine source, sodium iodide and potassium iodide are generally used for iodination reactions.
Iodination reactions using alkali iodides: Alkene and alkyne hydroiodination
Reaction details
Alkali iodide is combined with protonic acids, such as acetic acid or phosphoric acid, to carry out the hydroiodination of alkenes and alkynes. Details of these reactions are as follows below.
①Alkene hydroiodination
Reacting alkali iodide with a protonic acid will generate hydrogen iodide (HI) in situ. Following Markovnikov’s rule, the HI is added to the alkene’s double bond (electrophilic addition reaction), which produces secondary and tertiary iodoalkanes as reaction products.
Remarkably, using alkali iodides in this way tends to result in better yields than using HI directly. For example, Stone et al. succeeded in having KI and H3PO4 act on cyclohexane to synthesize iodocyclohexane at a high 88–90% yield3).
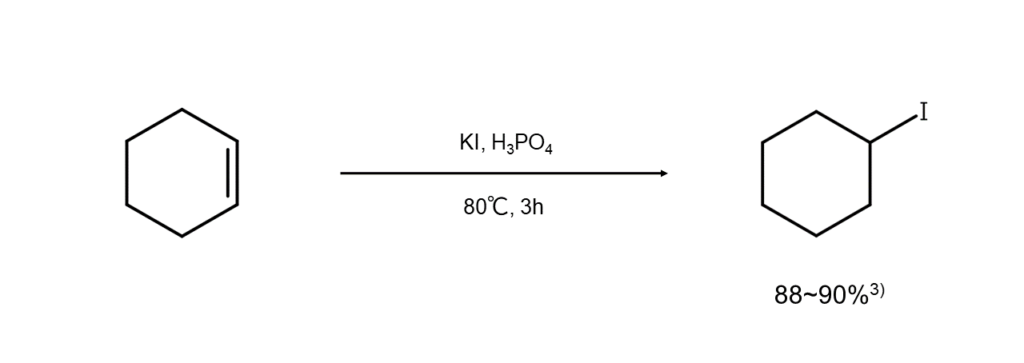
Using alkali iodides allows for both iodination and intramolecular cyclization. By converting an alkenylamine with a double bond within the beta–epsilon positions into a tosylamide and processing it with NaI and t-butyl hypochlorite ((CH3)3COCl), both iodination and intramolecular cyclization will occur, resulting in nitrogen ring compounds containing iodine functional groups.
There is an explanation offered regarding the reaction mechanism for the reaction above. First, t-butyl hypoiodite (CH3)3COI) is generated through halogen exchange. The resulting N-iodination produces N-iodosulfonamides, which attack the intramolecular olefin bonds and cause conversion into an iodonium ion intermediate. This is then supplemented with nitrogen atoms, forming a heterocycle. Minakata et al. report successfully generating high yields of three- to six-membered saturated N-heterocycles with total stereoselectivity using this reaction4).
Below are examples of alkene iodination reactions that also bring about intramolecular cyclization.
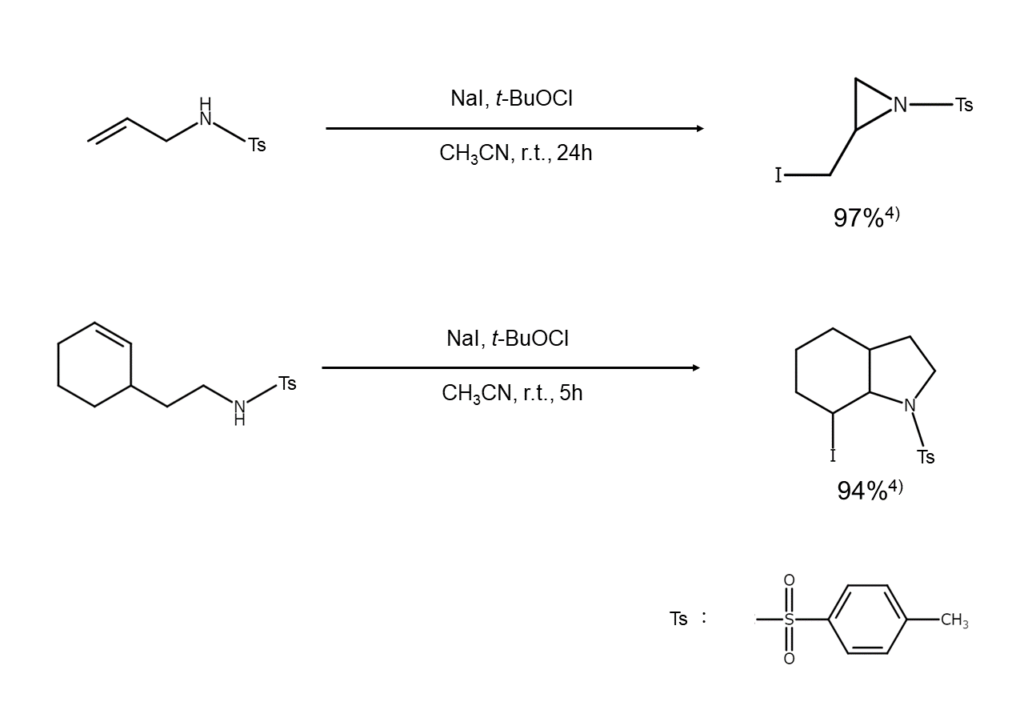
②Alkyne hydroiodination
The defining characteristic of alkyne hydroiodination is that it does not occur as readily as it does for alkenes. However, Michael-type additions easily occur for electron-deficient conjugated ketones such as ethynyl ketones, which enables high yields of beta-iodovinyl ketones5).
The stereochemistry of the above reaction products is determined by acid strength. Taniguchi et al. have reported that when reacting ketones containing a terminal acetylene group with sodium iodide, E-form can be primarily obtained in trifluoroacetic acid (TFA), and Z-form in acetic acid. Furthermore, when left for an extended period of time, the reaction mixture is also reported to gradually isomerize from Z-form to E-form6).
Below are examples of alkyne hydroiodination using alkali iodides.
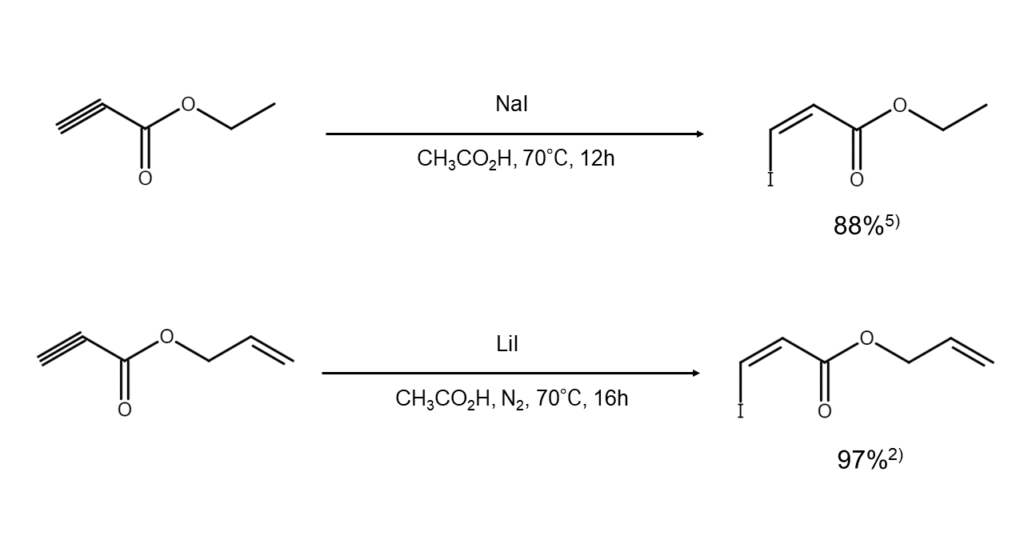
Iodination reactions using alkali iodides: Active aromatic compound iodination
Reaction details
The combination of an alkali iodide and a mild oxidant will iodinate active aromatic compounds, such as alkylbenzenes, phenols, and phenol ethers.
The reaction mechanism varies, including iodination resulting from hypoiodous acid generated in situ, as well as iodination that occurs via cation radical generation resulting from electron transfer. These reactions have long been used as effective iodination methods owing to the many excellent reactions they afford with favorable reaction conditions and high yields.
For example, using oxygen and a catalytic amount of nitrosonium salt (NO+BF4–) as oxidants allows for the iodination of active aromatic compounds under the presence of an alkali iodide or TFA. Note that since this reaction takes place through one-electron substrate oxidation from the nitrosonium ion (NO+), iodination is limited to arenes with a low redox potential7). Other known reactions include oxidation reactions such as those that use ammonium cerium(IV) nitrate8), which is a rare earth metal reagent, and iodination reactions that use potassium iodide and hydrogen peroxide9).
Below are examples of iodoarene synthesis using an alkali iodide with an oxidant.
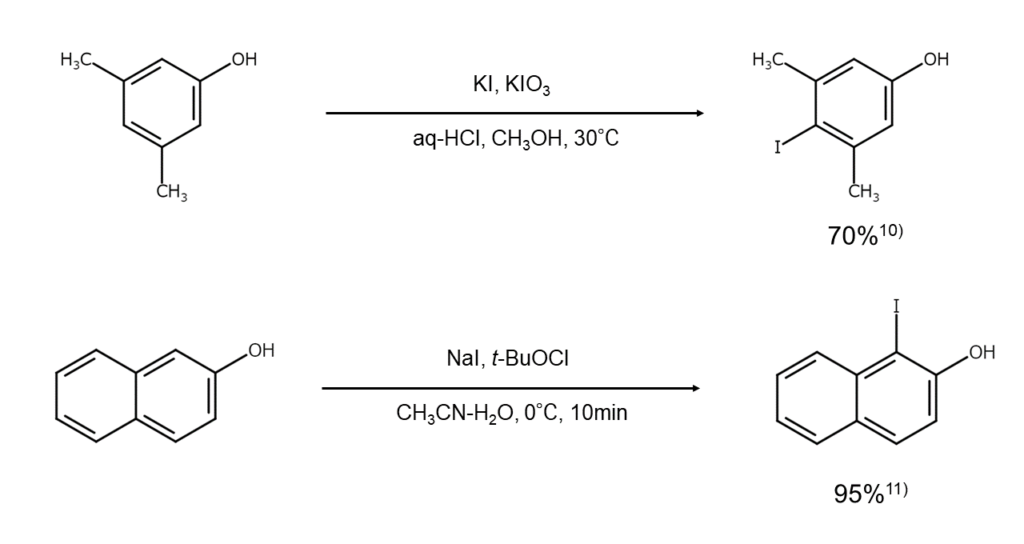
References
1) MANAC Inc., Research Laboratory, Suzuki, Hitomi (ed.), “Organic Syntheses of Bromine & Iodine Compounds”. Maruzen Publishing. (Japanese)
2) Ma, S., Lu, X. Tetrahedron Lett., 1990, 31, 7653.
3) Stone, H., Shechter, H. Org. Synth. Coll. Vol. IV, 543 (1963).
4) Minakata, S. Morino, Y. et al. Org. Lett., 2006, 8, 3335.
5) Marek, I., Meyer, C. et al. Org. Synth., 1997, 74, 194; Coll. Vol. IX, 510 (1998).
6) Taniguchi, M., Kobayashi, S. et al. Tetrahedron Lett., 1986, 27, 4763.
7) Rander, F. J. Org. Chem., 1988, 53, 3548.
8) Sugiyama, T. Bull. Soc. Chem. Jpn, 1981, 54, 2847.
9) Iskra, J., Stavber, S et al. Synthesis, 2004, 1869.
10) Hünig, S., Schwarz, H. Liebigs Ann. Chem., 1956, 599, 131.
11) Kometani, T., Watt, D. S. et al. Tetrahedron Lett. 1985, 26, 2043.