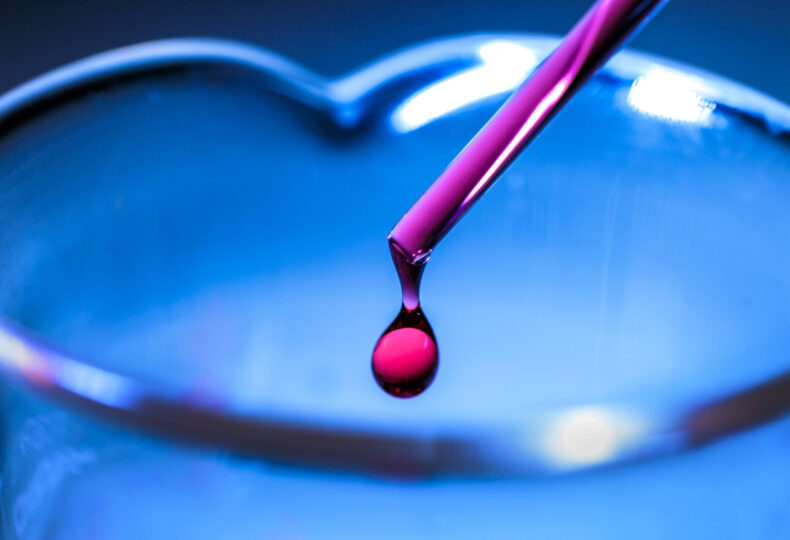
Iodoalkane synthesis from ether cleaving and other syntheses using alkali iodides: Iodination reactions using alkali iodides (3): Discussion series on bromination/iodination reactions 26
So far in this series, we have covered iodination reactions that use alkali iodides, commonly found as sodium iodide and potassium iodide. This article concludes our discussion of alkali iodides.
Our final alkali iodide discussion in this article will focus mainly on iodoalkane synthesis methods via ether or epoxide cleavage. Using these reactions requires understanding which functional group on which side of the ether oxygen will more easily undergo iodination. Let’s take a look into these and other reaction mechanism details.
In addition, we will also give a simple digest of other iodination reactions that could not fit into the series. Be sure not to miss any of the details.
contents
Iodination reactions using alkali iodides: Iodoalkane synthesis from ether cleaving
Iodoalkanes formed through nucleophilic substitution reactions
Setting an alkali iodide to act on an ether under the presence of an acid catalyst will cleave the ether bond and produce an iodoalkane. This reaction occurs due to iodine ions nucleophilically attacking the oxonium ion intermediates generated by ether oxygen protonation. The reaction mechanism is shown below.
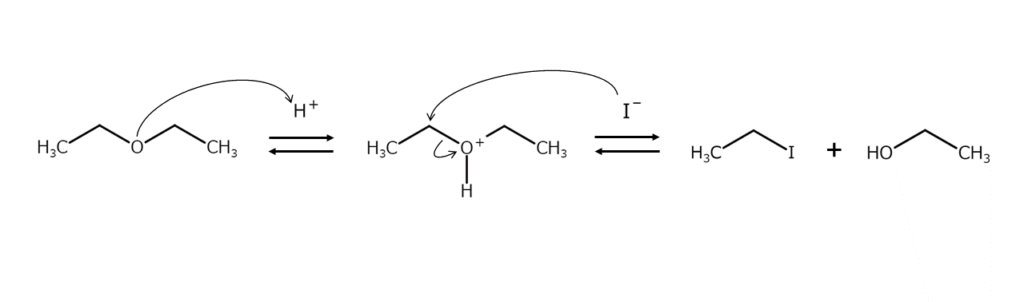
Just what kind of reaction products are produced through the above reaction? While iodoalkane mixtures can be obtained when asymmetric dialkyl ethers are cleaved, primary iodoalkanes are typically generated preferentially. Further, because allyl ethers, benzyl ethers, and tertiary alkyl ethers readily generate stable carbocations, iodination cleavage occurs preferentially on the alkyl group side of these compounds. In addition, alkyl ethers of phenols will always only give iodoalkanes.
NaI-phosphate is a commonly used reaction reagent with a broad scope of applications.2),3) However, for the cleavage of allyl ethers, which are highly reactive, methods that use KI in acetic acid or formic acid reportedly give better results.4) NaI-chlorotrimethylsilane, commonly used in the conversion of alcohols into iodoalkanes, can also be used in the iodination cleavage of ethers and esters.5)
The following illustrates ether iodination cleavage reaction examples.
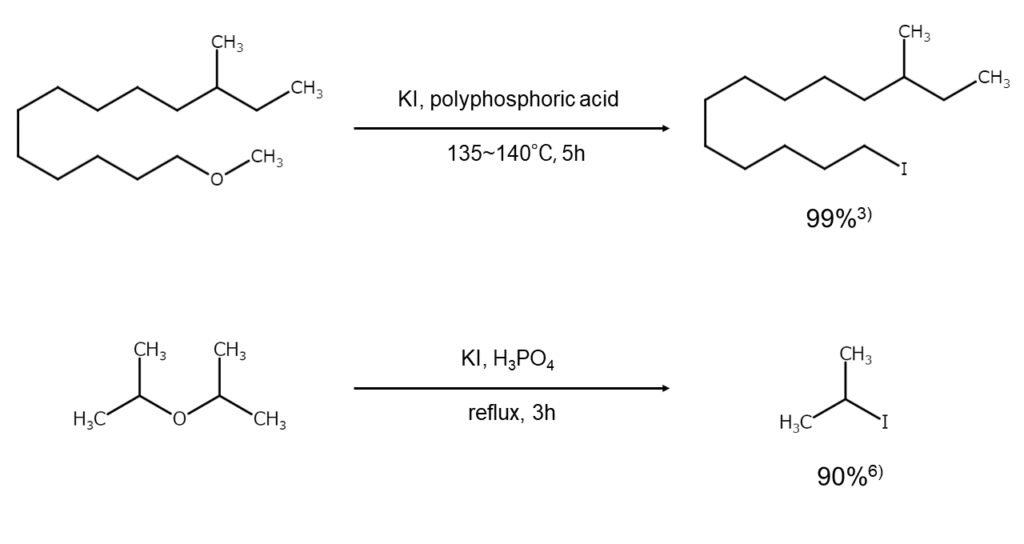
Epoxide reactants
Acetic acid solutions of LiI are highly effective for cleaving epoxides. However, caution is required as isomerization into a carbonyl compound easily occurs in aprotic solvents such as DMF or HMPA. In addition, epoxides will undergo cleavage with even mild Lewis acids, similar to MgI2, which results in an iodohydrin.7)
The following examples illustrate reactions with the iodination cleavage of epoxides.
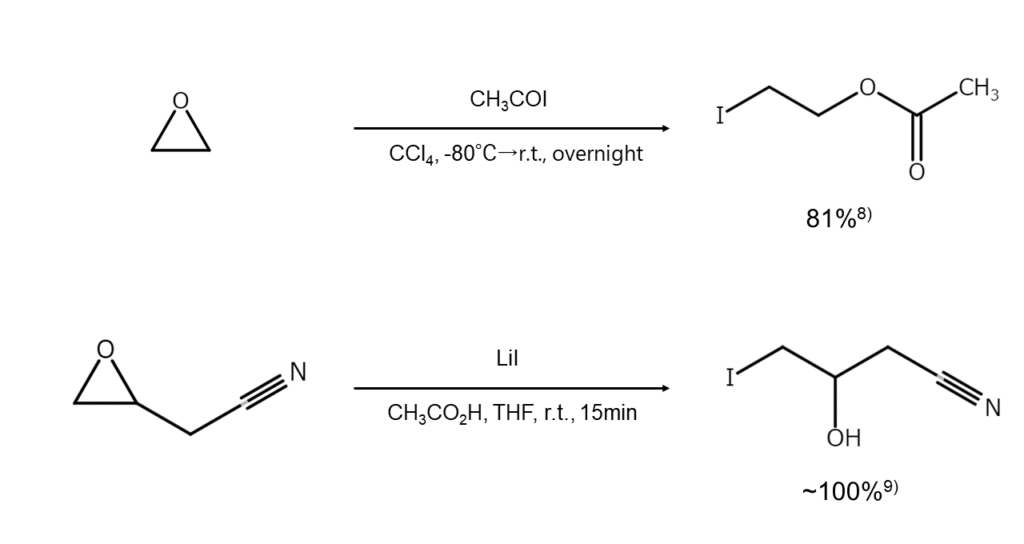
Iodination reactions using alkali iodides: Other syntheses using alkali iodides
Many other proposed iodination methods use alkali iodides. Here, we give a simple digest of some of the iodination methods that could not fit into this series for detailed review.
① Organic borane iodination cleavage, phenol iodination
NaI and chloramine-T are combined for the iodination cleavage of organic boranes and for phenol iodination. Reactions occur via iodine monochloride (ICl) generated in situ, which offers better results than ICI alone.10) Note that the ease of alkylborane cleavage is as follows, in order from easiest to most difficult: primary > secondary > tertiary.
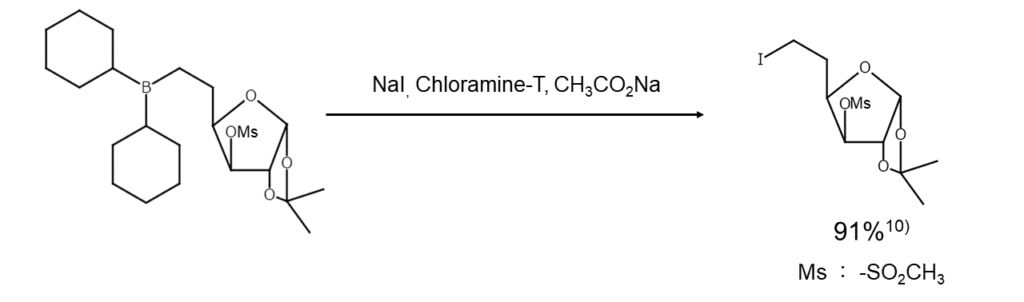
② Aromatic ring iodination
Hypoiodite ions generated from the action of NaI and aqueous NaOCl on a phenol in cooled methanol will easily iodinate aromatic rings. In terms of reaction products, depending on the reagent volume ratio, yields can be obtained at 80% for 4-iodophenol, 78% for 2,4-diiodophenol, and 90% for 2,4,6-triiodophenol.11)
③ Iodonio group iodination
In contrast to the general challenge of nucleophilically substituting a substituent on an olefin carbon, iodonio groups are easily substituted with iodine anions. Although the configuration will invert during substitution, introducing a sulfonyl group into the beta position will preserve the stereochemistry.12),13)
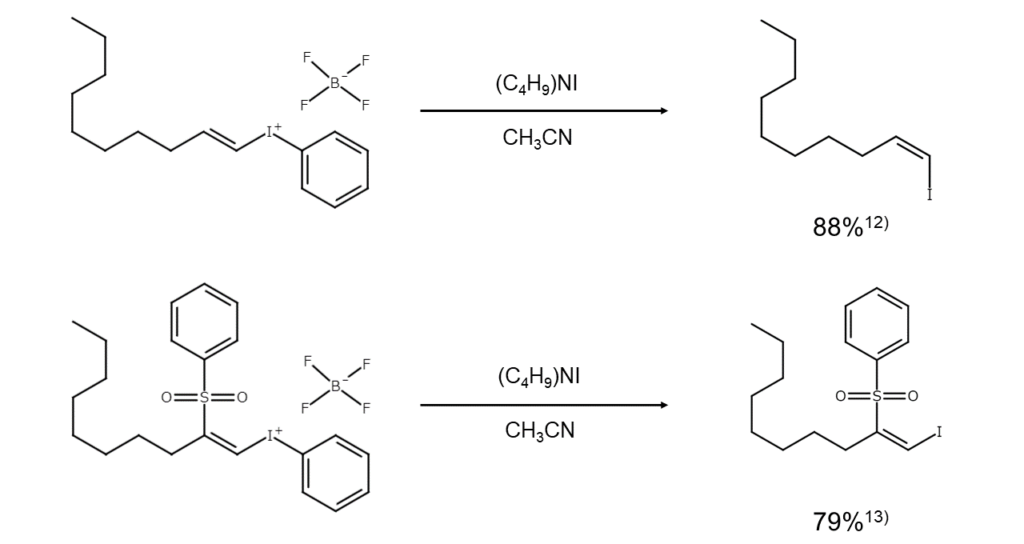
④ Selenone iodination cleavage
While iodine ions cannot be used to cleave sulfones, they rapidly cleave selenones, causing selenonyl groups to leave as selenenic acid ions and produce the corresponding iodide.14)
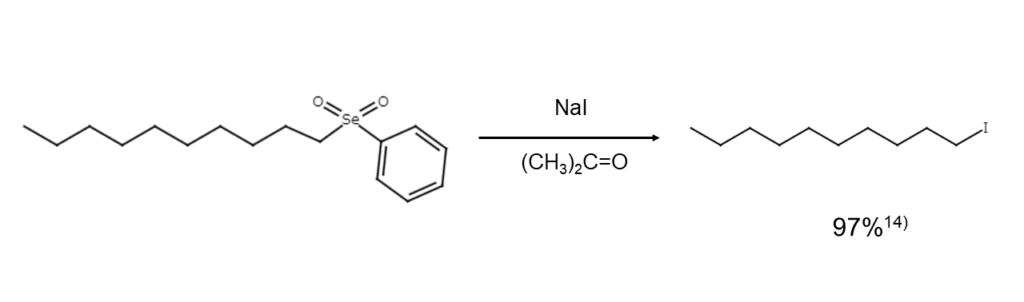
Column: The role of epoxides as insect pheromones
For chemists, the epoxides covered in this article are most strongly associated with compounds used for organic synthesis. However, epoxides also play a significant role in living organisms as well. One example of this is the role of epoxides as sex pheromones in moths.
Because moths are active at night, mating and egg-laying are carried out efficiently by means of information conveyed through scent rather than vision. Sex pheromones also constitute a form of olfactory information. Male moths detect sex pheromones secreted by female moths through olfactory receptors on the antennae, enabling them to search out females over a large area and mate.
There are two types of sex pheromones in moths: aliphatic pheromones (type I formed from acetyl-CoA) and hydrocarbon pheromones (type II formed from essential fatty acids).15) Among these, 60%–70% of moths with hydrocarbon sex pheromones use epoxides as sex pheromones. Research on the function of epoxidase in the biosynthesis of moth sex pheromones has been advancing in recent years.16)
There are many other examples of how epoxides are involved in a variety of ways in biological activities. We highly recommend for those interested to look more into this topic.
References
1) MANAC Inc., Research Laboratory, Suzuki, Hitomi (ed.), “Organic Syntheses of Bromine & Iodine Compounds”. Maruzen Publishing. (Japanese)
2) Stone, H., Shechter, H. Org. Synth. Coll. Vol. IV, 321 (1963).
3) Cope, A. C., Burrows, E. P. et al. J. Am. Chem. Soc., 1965, 87, 5452.
4) Mustafa, A., Sidky, M. M. et al. Liebigs Ann. Chem., 1967, 704, 182.
5) Olah, G. A., Narang, S. C. et al. J. Org. Chem., 1979, 44, 1247.
6) Stone, H., Shechter, H. J. Org. Chem., 1950, 15, 491.
7) Otsubo, K., Inanaga, J. et al. Tetrahedron Lett., 1987, 28, 4435.
8) Belsner K., Hoffmann, H. M. R. Synthesis, 1982, 239.
9) Bajwa, J. S., Anderson R. C. Tetrahedron Lett., 1991, 32, 3021.
10) Kabalka, G. W., Gooch, E. E. J. Org. Chem., 1981, 46, 2582.
11) Edgar, K. J., Falling, S. N. J. Org. Chem., 1990, 55, 5287.
12) Ochiai, M., Oshima, K. et al. J. Am. Chem. Soc., 1991, 113, 7059.
13) Ochiai, M., Oshima, K. et al. Tetrahedron Lett., 1991, 32, 7711.
14) Krief, A., Dumont, W. et al. J. Chem. Soc., Chem. Commun., 1985, 571.
15) Ando, T., Inomata, S. et al. Topics in Current Chemistry, 2004, 239, 51.
16) Shimizu, N., et al. “Konchuu no Feromon 2: Shibousan ni Yurai Suru Feromon no Seigousei to Juyou Kikou” [“Insect Pheromones 2: Biosynthesis and Receptor Mechanisms for Pheromones Derived From Fatty Acids”],
<https://www.jstage.jst.go.jp/article/kagakutoseibutsu/57/12/57_571208/_pdf> (Japanese)