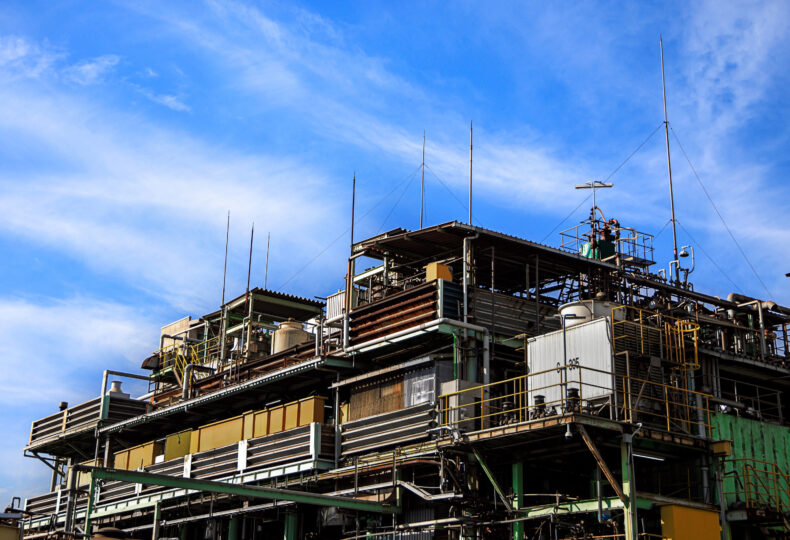
Iodine addition to alkenes: Aliphatic compound iodination reactions (2): Discussion series on bromination/iodination reactions 19
In this series, we discuss bromination and iodination reactions, specialties of MANAC. Continuing from the previous article, we are reviewing aliphatic compound iodination reactions with elemental iodine.
In this article, we look into iodine addition reactions for alkenes.
Similar to the alkane reactions introduced in the last article, alkene iodination reactions are rather inefficient. This fact makes it all the more valuable to know the principles and suitable reaction conditions behind these reactions.
Be sure to read to the end of the article as we again dive into the details of reaction mechanisms and example reactions.
■ What you can learn from this article ✔ Addition reactions of elemental iodine to alkenes exhibit high reversibility, progress slowly, and produce unstable products, limiting their applications. ✔ Ionic mechanisms proceed using polar solvents or catalysts, allowing for the addition of atoms other than iodine. Radical mechanisms rely on light or heat but are prone to side reactions. ✔ By combining elemental iodine with other reagents, it is possible to obtain products with structures that are otherwise unattainable, such as Markovnikov alkyl iodides or anti-Markovnikov alkyl iodides. ■ Recommended Articles ・ Alkyne iodination: Aliphatic compound iodination reactions (3): Discussion series on bromination/iodination reactions 20 ・ Bromination reactions with hydrogen bromide (additions to alkenes/alkynes): Hydrogen bromide (3): Discussion series on bromination/iodination reactions 36
contents
Aliphatic compound iodination reactions with elemental iodine: Iodine addition to alkenes
A synthesis method with poor efficiency and limited usage
Elemental iodine addition reactions for alkenes have high reversibility, and so reactions can only progress slowly. Further, diiodoalkane, generated as a reaction product, is generally unstable and easily degrades as it releases iodine or hydrogen iodide (HI) at room temperature. Consequently, this reaction is currently limited in its scope of application.
Two reaction mechanism types: Ionic mechanisms and radical mechanisms
The single term “alkene” indeed encompasses a wide range of structures. It then goes without saying that the ease with which addition reactions occur differs between structures. In general, the following order is considered conducive to facilitating iodine addition reactions.
CH2=CHCH3 > CH2=CHCH2OH > CH2=CH2 > CH2=CHCH2OCOCH3 > CH2=CHCH2Br
There are two types of elemental iodine addition reactions for alkenes: ionic mechanisms and radical mechanisms. Details are given on each of these below.
①Ionic mechanisms
Ionic mechanisms occur through the generation of ionic species resulting from complex formation between iodine molecules in a polar solvent.
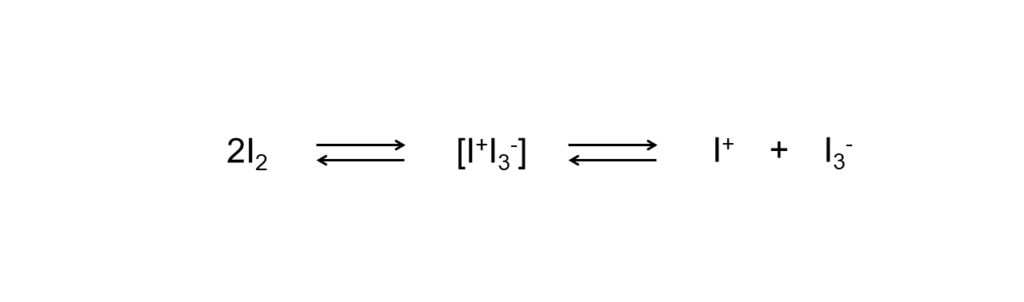
These reactions do not readily occur with iodine alone, so catalysts (such as an acid catalyst or a catalytic solid surface) are generally used. It is also known that brown solutions (acetic acid, alcohols, nitrobenzene) facilitate faster additions over purple solutions (carbon tetrachloride, chloroform, carbon disulfide, hexane).
②Radical mechanisms
Radical mechanisms progress in a chain through the occurrence of iodine atoms resulting from heat application or photoexcitation in a gaseous or polar solvent.
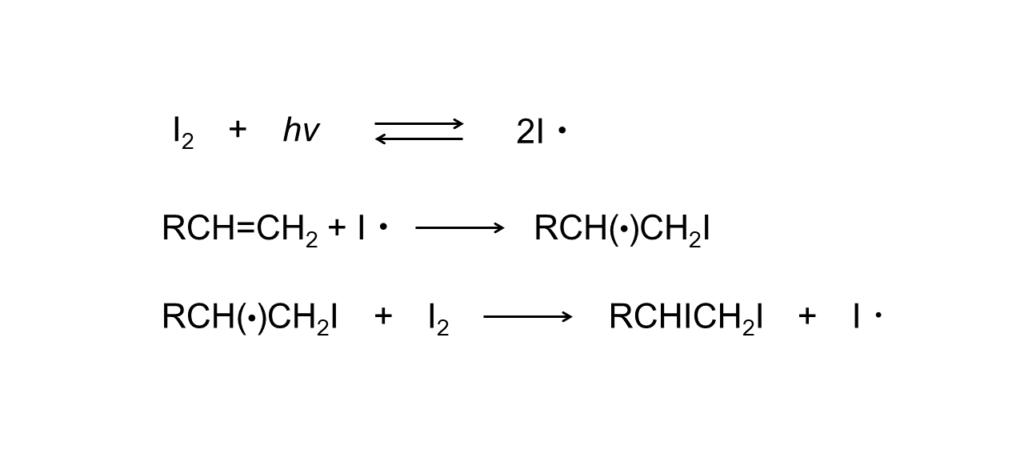
In the case of photoexcitation, reactions are generally conducted at a temperature between -50°C and -90°C using a light wavelength between 4200 nm and 4500 nm. However, since quantum yields are highly dependent on alkene structures, conditions must be considered separately for each reaction.
Another factor that cannot be ignored is the impact of side reactions. When using ultraviolet light or conducting reactions at room temperature, the polymerization of alkenes and the photodegradation of reaction products take precedence, and iodine addition reaction products cannot be readily achieved.
For example, when setting elemental iodine to act on styrene or butadiene at room temperature, a polymerization reaction begins instead of an addition reaction. When setting elemental iodine to act on allyl alcohol, an addition reaction occurs and 2,3-diiodopropanol crystals separate. However, these crystals rapidly degrade into a dark-colored substance.
Ionic mechanisms: Possible additions other than iodine atoms
Ionic mechanisms enable the obtainment of iodoalkanes with one of many different functional groups depending on how reaction conditions are arranged.
①Introducing functional groups corresponding to methanol, acetic acid, or other nucleophilic species
Suppose that elemental iodine is set to act on an alkene under the presence of a nucleophilic species in a polar solvent. Doing so adds one iodine and generates a cyclic iodonium ion intermediate, which is then captured by the nucleophilic species. This produces an iodoalkane with a functional group corresponding to the nucleophilic species.
Methanol, acetic acid, and alkylamine are commonly used as nucleophilic species.
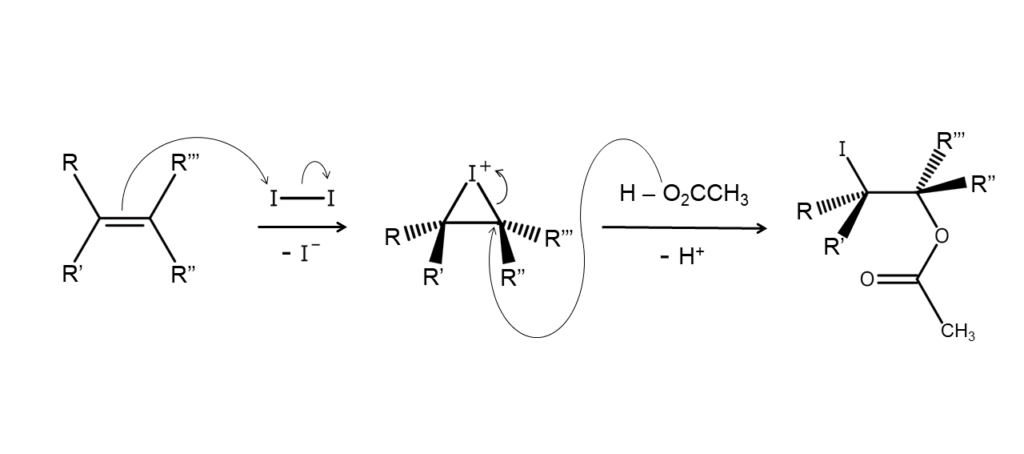
②Introducing functional groups corresponding to hypoiodous acid and its derivatives
When elemental iodine is set to act on an alkene using water, alcohol, or carboxylic acid as a solvent, hypoiodous acid (HOI), the alkyl derivative of HOI (ROI), and the acyl derivative of HOI (RCOOI) are each generated within the system. These subject alkenes to iodohydroxylation, iodoalkoxylation, or iodoacylation, which generates the corresponding iodide (such as iodohydrin or its derivative).
As is the case with ① directly above, this reaction also occurs via an iodonium ion intermediate. The HOI, ROI, and RCOOI in the system function both as nucleophilic species that attack the iodonium ion intermediates while also readily facilitating the generation of the same iodonium ion intermediates.
There are also many cases when synthesizing iodohydrin derivatives where concurrent use of a mild oxidant or acid catalyst produces favorable results.
The following are examples of reactions that use water or methanol as a solvent.
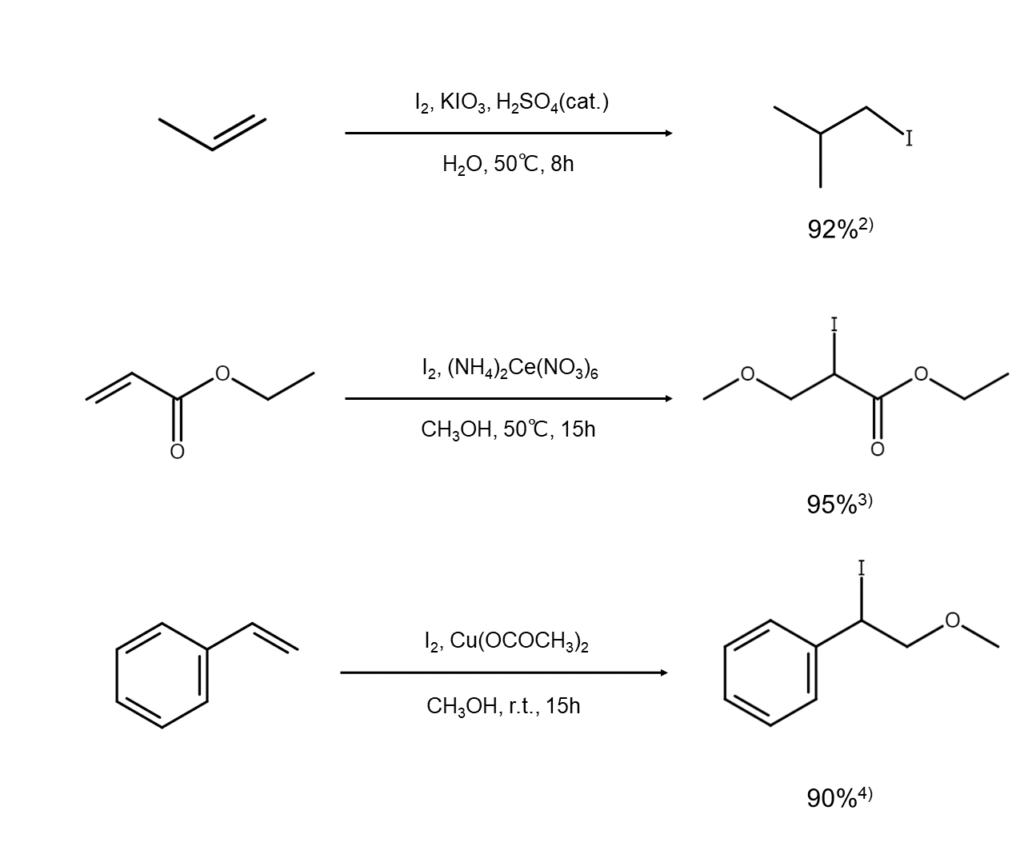
③Introducing fluorine as a functional group
Using the high-valent iodine reagent 4-iodotoluene difluoride (4-CH3C6H4IF2) together with elemental iodine facilitates iodofluorination, which then introduces a fluorine functional group. This reaction is presumed to occur through fluorine anions (F–) acting as nucleophilic species by attacking iodonium ion intermediates.
The following illustrates an example of this reaction.
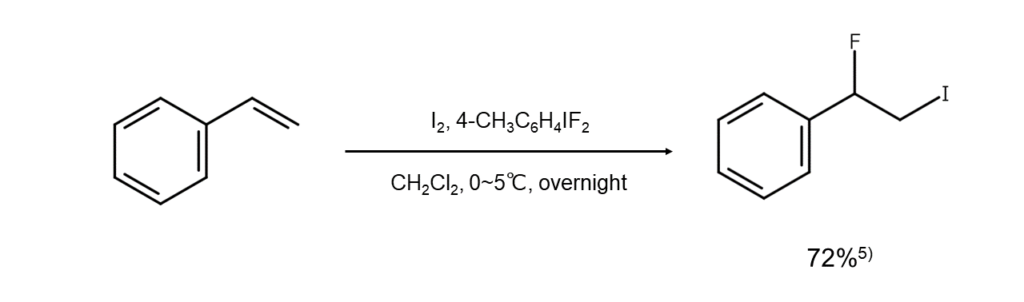
Ionic mechanisms: Heterocyclic compound synthesis possible with an olefin bond within the gamma–epsilon positions
When elemental iodine is reacted with an alcohol, thiol, or carboxylic acid, which have olefin bonds within the gamma-epsilon positions, the generated cyclic iodonium ion intermediate undergoes an SN2 attack by an intramolecular heteroelement functional group (such as a hydroxy group). This results in a high yield of the corresponding cyclic ether, cyclic sulfide, or lactone.
In these types of reactions, complex skeletons can be created in one step by effectively leveraging the substrate structure. These reactions are, therefore, widely used to synthesize heterocyclic compounds related to pharmaceuticals and natural products. The following are examples of common reactions (to learn about other reaction examples, see references 7–10 listed at the end of this article).
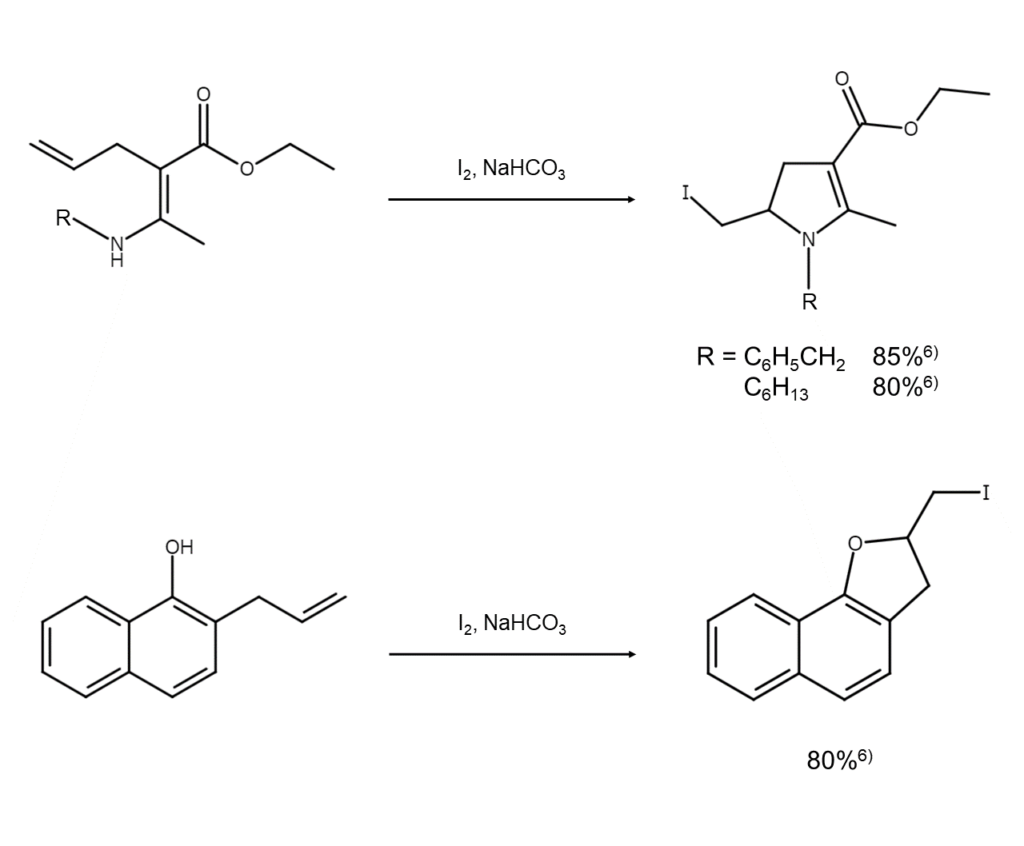
These heterocyclic compounds, which have an iodine functional group on the alkyl side chain, are generally highly reactive, easily emitting HI and undergoing polymerization when heated or subjected to long-term storage. Correctly managing these compounds is key to preventing polymerization.
Skin contact must also be avoided, as many heterocyclic compounds cause severe skin irritation. Exercise adequate caution when handling these compounds.
Column: Anti-Markovnikov additions possible with the use of hydroboration and elemental iodine
Combining elemental iodine with other reagents enables the creation of reaction products with structures that normally cannot be obtained. Let’s go over an example.
As shown below, adding HI to an alkene typically results in a Markovnikov alkyl iodide via a more stable carbocation intermediate.
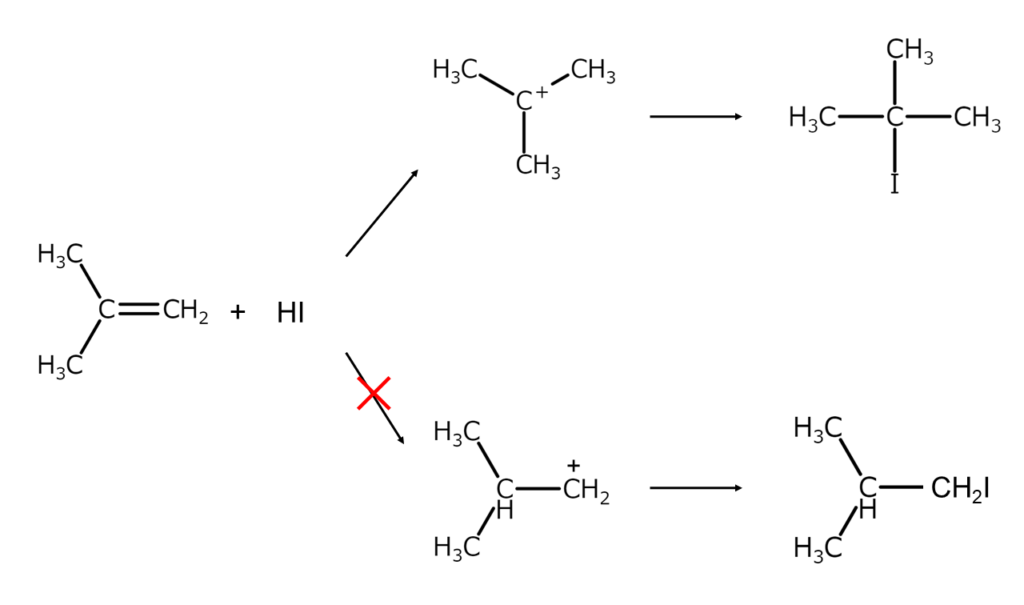
If this is the case, what is needed to obtain an anti-Markovnikov alkyl iodide? The solution to this question is hydroboration and elemental iodine.
After hydroborating an alkene with a borane, an anti-Markovnikov product can be obtained by processing the resulting trialkylborane (R3B) with elemental iodine (iodinolysis). Because organoborons such as R3B generally react slowly with elemental iodine, they are usually first converted into a boronate by setting a strong alkali, such as sodium hydroxide or sodium methoxide, to act on them. The resulting product is then cleaved using elemental iodine. The reaction formula is shown below.
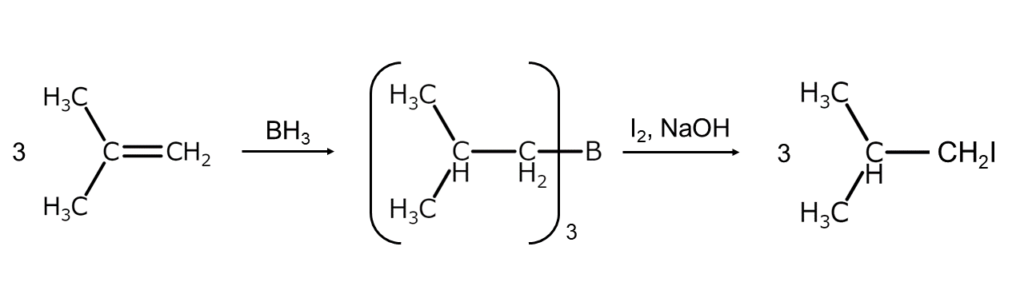
Be sure to try this reaction when an anti-Markovnikov addition compound is needed.
References
1) MANAC Inc., Research Laboratory, Suzuki, Hitomi (ed.), “Organic Syntheses of Bromine & Iodine Compounds”. Maruzen Publishing. (Japanese)
2) Cornforth, J. W., Green, D. T. J. Chem. Soc. C, 1970, 846.
3) Horiuchi, C. A., Ochiai, K. et al. Chem. Lett., 1994, 185.
4) Georgoulis, C., Valery, J. M. Synthesis, 1978, 402.
5) Conte, P., Panunzi, B. et al. Tetrahedron Lett., 2006, 47, 273.
6) Pacote, C. G., de Carvalho, B. S. et al. Synthesis, 2009, 3963.
7) Dowle, M. D., Davies, D. I. Chem. Soc. Rev., 1979, 8, 171.
8) Cardillo, G., Orena, M. Tetrahedron, 1990, 46, 3321.
9) Robin, S., Rousseau, G. Tetrahedron, 1998, 54, 13681.
10) Ranganathan, S., Muraleedharan, N. K. et al. Tetrahedron, 2004, 60, 5273.